Apicomplexa
Sporozoa
Jan lapeta and Victoria Morin-Adeline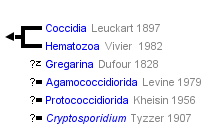


This tree diagram shows the relationships between several groups of organisms.
The root of the current tree connects the organisms featured in this tree to their containing group and the rest of the Tree of Life. The basal branching point in the tree represents the ancestor of the other groups in the tree. This ancestor diversified over time into several descendent subgroups, which are represented as internal nodes and terminal taxa to the right.
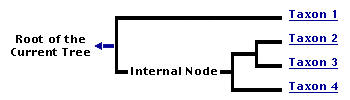
You can click on the root to travel down the Tree of Life all the way to the root of all Life, and you can click on the names of descendent subgroups to travel up the Tree of Life all the way to individual species.
For more information on ToL tree formatting, please see Interpreting the Tree or Classification. To learn more about phylogenetic trees, please visit our Phylogenetic Biology pages.
close boxIntroduction
Apicomplexa contains a large assortment of single celled eukaryotic organisms, known to parasitise vertebrates (including humans) and invertebrate hosts. It is estimated that out of a probable 1.2 - 10 million apicomplexan species, only about 0.1% have been named and described to date (Adl et al., 2007). As a group, apicomplexans are complex organisms that exhibit a wide variety of morphological shapes depending on their genus and lifecycle stage. They are also regarded to be reasonably host specific, inhabiting a wide array of terrestrial and marine environments depending on their host niche environments.
This group of obligate parasites evolved from a free-living photosynthetic ancestor (=chromerids) and it is closely related to free living single-cell predators (=colpodellids).

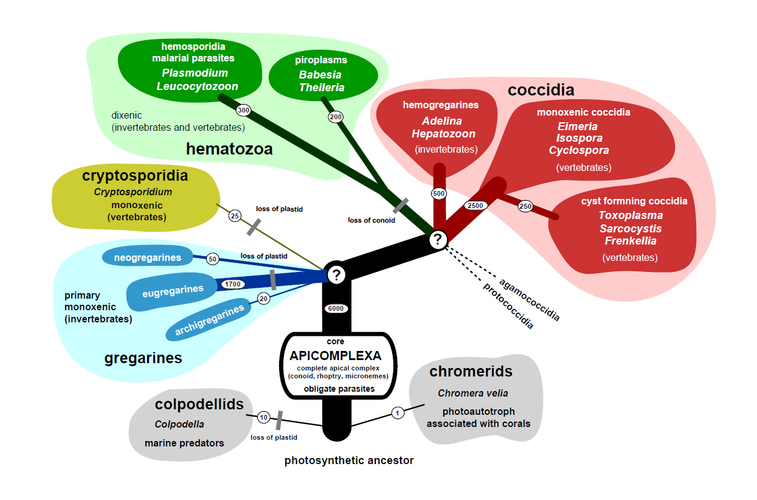
Hypothetical tree of Apicomplexa. Three principal parasitic groups are coloured and their life cycle indicated, as well as Cryptosporidium that likely emerged from within gregarines. Numbers on branches and thickness indicates diversity (i.e. named species). © 2011 Jan Slapeta
While a large portion of the apicomplexans are known parasites of virtually all vetrebrates including humans, they are equally diverse in both marine and terrestrial invertebrates. In fact, we are sure there is at least one unique apicomplexan parasite per any invertebrate or vertebrate.
The most eminent of the apicomplexan parasites of humans are Plasmodium spp. from the Hematozoa clade, which cause malaria responsible for >1 million deaths annually (Manguin et al., 2010; Mackintosh et al., 2004). Another parasite, Toxoplasma gondii is present in aproximatelly 30% of humans worldwide as brain cysts causing no apparent disease; however, in immunonaive pregnat women it may cause serious disease afecting her foetus. Apicomplexan diseases of domestic animals are associated mainly with farmed animals and are renowned for the large economic costs incurred by the agricultural industry. Of these Eimeria spp. is known to be responsible for a $1.5 billion loss to the poultry broiler industry each year worldwide (Sharman et al., 2010). Similarly, Neospora caninum is linked to losses in the dairy as well as beef industry (Trees et al., 1999).
Parasitism in wildlife populations has been recorded as frequently as in domestic populations. However, in a wild setting, parasitism seems to act to reduce vigor and alter behaviour of the host rather than cause significant disease and losses (Hamilton and Zuk, 1982, Horak et al., 2004, Bouma et al., 2006, Morin-Adeline et al., 2011). How parasite burden and pathology in captive animals translate into the wild is of much interest, especially when large resources are being placed into captive breeding programs of endangered species.
Characteristics
The use of electron microscopes has led to the discovery of unique intracellular structures that led to the description of Apicomplexa as well as their sub-grouping (Levine, 1973).
Ultrastructural Characteristics of Apicomplexa
- Polar rings
- Conoid
- Micronemes
- Rhoptries
- Dense granules
- Pellicle (i.e. inner membrane complex)
- Micropores
The apical complex is the flag trait required for classification as Apicomplexa (Lee et al., 2000, Levine, 1973). It is a set of ultrastructurally and functionally homologous components found at the anterior end of certain stages, most notably the infective stages, displacing the nucleus and mitochondria towards the posterior end (Aikawa et al., 1978). The structural (cytoskeletal) components of the apical complex are known as the conoid, polar rings and sub-pellicular microtubulles. Three types of distinct secretory organelles are typically present: rhoptries, micronemes and dense granules. Rhoptries and micronemens are always associated with the anterior end, while dense granules are often in the posterior end as well.
The feature that unifies Apicomplexa with other alveolates (Alveolata) is a distinct membrane complex that envelopes all infective stages. The outermost membrane part is called plasmalema. The inner part is composed of two membranes runnig parrallel to each other forming the flattened inner membrane complex (a.k.a. alveoli). The pellicle is interrupted at the anterior end by the polar rings associated with the apical complex and a single or several micropores. Besides the above, they are comprised of endosymbiontic derived organelles (mitochondrion, apicoplast), universal eukaryotic organelles (nucleus, endoplasmic reticulum, Golgi apparatus, ribosomes), and specific compartments (i.e. acidocalcisomes), all enclosed by a complex membranous structure termed the pellicle.

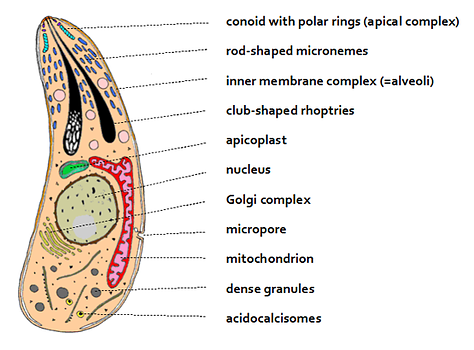
Schematic drawing of a typical Apicomplexa. © 2011 Jan Slapeta
Although all apicomplexans contain rhoptries, micronemes and dense granules, the number and general shape of these secretory organelles alter between different groups (Blackman and Bannister, 2001, Leander, 2007a). For instance, most coccidia and hematozoa are known to possess two rhoptries in their merozoites (Bannister and Mitchell, 1989), however a few others have been documented to contain more, an example being the triplet found in Babesia caballi (Kawai et al., 1999). Nonetheless, the proteins secreted from these secretory structures are associated with initiation and regulation of the host cell invasion process, controlled by the secretion of a vast array of proteins (Carruthers, 1999, Bannister and Mitchell, 1989, Proellocks et al., 2010). However, the conoid is not present in the hematozoan group (e.g. Plasmodium spp.) known by some authors as Aconoidasida.
In Toxoplasma gondii and presumably other apicomplexans, the conoid is made of tubulin fibres arranged in a unique manner and it has the potential to expand and reduce in a Ca2+ dependent manner to produce some form of movement (Hu et al., 2002). Therefore, in addition to invasion of host cells the apical complex may have a vital role to play in parasite orientation, support and movement prior to and after invasion. In gregarines, the conoid structure is often not observed and is replaced instead with a homologous mouth piece known as a mucron that attaches to host cell extracellularly for feeding (Dyson et al., 1994, Leander, 2007a). It has been suggested that these organisms are dependent on the attachment of the mucron to the host cell for structural support (Leander, 2007b).
Apicoplast
The apicoplast is a remnant non-photosynthetic plastid characteristic of apicomplexans consisting of a 35kb circular genome (Wilson et al., 1996). Discovery and recognition of this organelle occurred as recently as fifteen years ago in the genus Plasmodium with the aid of in situ hybridisation techniques to a 16S plastid rRNA gene (McFadden et al., 1996). Since then much emphasis has been placed on research of this organelle due to its potential to act as a drug target for the treatment of diseases caused by apicomplexan parasites (Williamson et al., 2002). It is hypothesised that all photosynthesising organelles within higher organisms originated from a primary endosynbiont process that initiated after a eukaryote engulfed and enslaved a photosynthesising cyanobacterium, resulting in a photosynthesising eukaryote (Green, 2011). During the course of time, another (aka secondary) endosymbiont event is thought to have occurred whereby the photosynthesising eukaryote was engulfed by a heterotrophic organism to give rise to the multiple membrane plastid (Cavalier-Smith, 1999). It was later confirmed that this sequence of events seemed likely as the origin of the apicoplast in apicomplexan parasites, and with the discovery of Chromera velia in 2008, the origin of the apicoplast has been traced back to red algal origins despite being significantly reduced in nature (Fast et al., 2001, Janouskovec et al., 2010, Waller and McFadden, 2005).
Plastid rRNA gene analyses performed on multiple apicomplexans have confirmed that all apicomplexans seem to posses the plastid-like structure with the exception of Cryptosporidium spp. and Gregarina niphandrodes - a eugregarine species (Lang-Unnasch et al., 1998, Zhu et al., 2000, Toso and Omoto, 2007). The conservation of this relic structure within the majority of apicomplexans implies that apicoplasts still carry out an important function required for parasite survival (Lang-Unnasch et al., 1998). The apicoplast has been identified as critical for parasite survival as it is responsible for the anabolic FASII and DOXP pathways, representing fatty acid synthesis and isoprenoid synthesis respectively, both of which have been deemed critical to parasite survival (Brooks et al., 2010).
Invasion of Host Cells
Invasion of host cells by apicomplexan parasites is highly conserved between related protozoa, with the possible exception of Theileria (Shaw, 2003). Attachment to the host cell is initiated via interaction of its surface proteins to the host cell's plasma membrane, an example being the SAG glycoprotein in Toxoplasma gondii (Grimwood and Smith, 1996). The apical region of the infecting parasite (zoite) then connects to the host cell forming a depression in the cell plasma membrane, taking the shape of the zoite while creating a condensed, electron-dense area at the point of attachment (Aikawa et al., 1978). Rhoptry ducts extend from the apical complex and through the junction formed between the two cells (Aikawa et al., 1978). This step is followed by the release of vast array proteins from the microneme and rhoptry of the apical complex which have the capacity to induce formation of the protective parasitophorous vacuole (PV) that surrounds the parasite once inside the host cell (Bannister and Mitchell, 1989). The actual invasion of the parasite is mediated by the formation of a moving junction around the infecting parasite which is so named because it moves along the length of the parasite resulting in the engulfment of the parasite within the host cell (Besteiro et al., 2009).
General Life Cycle
The basic life cycle of the apicomplexan parasite is typically complicated. Generally, there are four transformations to complete the cycle: the zygote, a sporozoite, a merozoite, and a gametic stages. The typical lifecycle of apicomplexa is well established as they all undergo a series of asexual and sexual reproduction involving either one or two hosts whereby a diploid zygote is formed by the union of gametes. It then undergoes a meiotic division to form infective haploid sporozoites. Within the body, sporozoites are thought to target specific cells by the use of a range of molecular armaments which include surface adhesions such as parasite surface proteins and binding antigens to enter host cells (Baum et al., 2008). The sporozoites then transform to form merozoites within the host cells, followed by several additional generations of asexual division to produce more merozoites and eventually sexual gametes are produced leading to fertilization and the production of a diploid zygote followed by formation of infective diploid sporozoites (Levine, 1973; Lee et al., 2000).
The fine details within this life cycle vary within different apicomplexan groups. For example in coccidian parasites, access to atmospheric oxygen levels induces sporulation of the zygote to form haploid sporozoites, so oocysts are released into the environment non-infectious (Levine, 1973). In contrast, oocyst formation and sporulation in haematozoan parasites occurs within the gut cells of an invertebrate host and the released sporozoites are injected into a new host upon invertebrate feeding (Krotoski, 1985). The presence of an additional dormant phase in the Plasmodium vivax life cycle is also well established and is associated with the relapse of diseases seen during infections. Few apicomplexans have been documented to having this phase, but it is suggested that it may be a common occurrence in many related apicomplexans which have been overlooked (Markus, 2010). Furthermore, differences between species arise in the localization of each stage. For example, in the Plasmodium spp. it is well understood that merozoites target erythrocytes while sporozotes target hepatocytes (Baum et al., 2008). This is contrary to the merozoites in the genera Eimeria and Isospora which are located in the intestinal epithelial cells of the villi (Fernando, 1974, Morin-Adeline et al., 2011).
The life cycles of different groups vary in terms of the number of hosts involved and the type of cellular invasion. While coccidians and gregarines are known to parasitise a single host and an environmental stage to complete their lifecycle, haematozoans have to make use to two hosts with no stages occurring in the environment (Cox, 2010, Lee et al., 2000, Levine, 1973, Moller and Arriero, 2008, Prensier et al., 2008). Moreover, while gregarines infect only invertebrate hosts and coccidia infect only vertebrate hosts, haematozoans make use of both invertibrate and vertebrate hosts (Cox, 2010, Lee et al., 2000, Prensier et al., 2008).
Discussion of Phylogenetic Relationships
The apicompexans, dinoflagellates and the ciliates are classified as Alveolata, along with the newly discovered Chromera velia, an organism that shares many similarities with the putative ancestor of Apicomplexa (Lee et al., 2000, Moore et al., 2008). Analysis of the small subunit rRNA has confirmed that apicomplexans are more closely related to dinoflagellates then to ciliates (Van de Peer and De Wachter, 1997, Fast et al., 2002). However more recently, investigations of plastid genes has uncovered a more intimate relationship with the phylum Chromerida (Moore et al., 2008). Furthermore, 18 plastid genes were found in common between the alveolate lineage and red algae, confirming the red algal roots of apicomplexa (Janouskovec et al., 2010).
Phylum: Apicomplexa Levine, 1970 (syn. Sporozoa Leucart, 1879)
Traditionally the grouping called Apicomplexa comprises obligate parasites. Nevertheless, the phylum Apicomplexa Levine, 1970 was conceived to include free living marine flagellates of the genus Perkinsus and the traditional Sporozoa Leucart, 1879 emended to include gregarines and coccidia only, therefore omitting hematozoa (Levine, 1970). Perkinsus spp. are no longer considered Apicomplexa, therefore Sporozoa and Apicomplexa are equally good descriptors for the group. Apicomplexa has become frequent in recent literature but it is not used exclusively. We consider both terms synonyms, but use Apicomplexa throughout the text.
One of the distinct characters of Apicomplexa is their parasitic life style. Therefore, free-living predatory flagellates classified as Colpodellida Cavalier-Smith, 1993 are not included within Apicomplexa. Colpodellida Cavalier-Smith, 1993 and Chromerida Moore et al., 2008 are considered independent groups.
Apicomplexa comprise five principal groups commonly known as 'gregarines', 'haemogregarines', 'coccidia', 'malarial parasites' and 'piroplasms'. Besides these dominant five groups, there is a myriad of small transitional groups or species, for example the cryptosporidia forming an independent group.
Formally Apicomplexa is split into three principal groups; the Coccidia (=coccidia , haemogregarines), Gregarina (=gregarines) and Hematozoa (=malarial parasites, piroplasms), all of which are obligate parasites. It has been postulated that before any morphological characteristic is utilised as the basis of taxonomical classification, its association with that particular group of organism has to be confirmed with clade analysis (Clopton, 2009, Morrison, 2009). The lack of such support for morphological traits, and additional errors associated with inappropriate representation of taxa and misuse of genetic analysis tools in the classification of organisms within the three different groups has complicated this field over the years (Morrison, 2009). Recently, much emphasis has been placed on identification of clade-supported traits in an attempt to improve classification criteria (Clopton, 2009, Jirku et al., 2008, Imam, 2009, Adl et al., 2007).
Recently, Apicomplexa became the home for one of the longest-standing protist enigmata - Nephromyces (Saffo et. al., 2010). Nephromyces are endosymbiotic marine protists, whose classification was uncertain ever since they were described in the 19th century. These protists inhabit the lumen of a ductless, urate- and calcium oxalate-rich organ of uncertain function (a.k.a. renal sac), in molgulid ascidian tunicates (Urochordata: Chordata). These protists are ubiquitous nonhereditary symbionts, transmitted horizontally to new hosts. Using rDNA and some apicomplexa-like ultrastructure, Nephromyces form a monophyletic group most closely related to Cardiosporidium cionae, a parasite found in pericardial bodies of an ascidian, Ciona intestinalis (Ciancio et al., 2008; Saffo et al., 2010). Together these protist form a group within Hematozoa (Aconoasida). These examples indicate how little we know about the diversity and habitats that Apicomplexa were able to invade and establish themselves in. No doubt more surprises are still out there.
References
Adl, S. M., Leander, B. S., Simpson, A. G. B., Archibald, J. M., Anderson, O. R., Bass, D., Bowser, S. S., Brugerolle, G., Farmer, M. A., Karpov, S., Kolisko, M., Lane, C. E., Lodge, D. J., Mann, D. G., Meisterfeld, R., Mendoza, L., Moestrup, O., Mozley-Standridge, S. E., Smirnov, A. V. & Spiegel, F. 2007. Diversity, nomenclature, and taxonomy of protists. Systematic Biology, 56, 684-689.
Aikawa, M., Miller, L. H., Johnson, J. & Rabbege, J. 1978. Erythrocyte entry by malarial parasites- moving junction between erythrocyte and parasite Journal of Cell Biology, 77, 72-82.
Bannister, L. H. & Mitchell, G. H. 1989. The fine-structure of secretion by Plasmodium knowlesi merozoites during red-cell invasion Journal of Protozoology, 36, 362-367.
Baum, J., Gilberger, T. W., Frischknecht, F. & Meissner, M. 2008. Host-cell invasion by malaria parasites: insights from Plasmodium and Toxoplasma. Trends in Parasitology, 24, 557-563.
Besteiro, S., Michelin, A., Poncet, J., Dubremetz, J. F. & Lebrun, M. 2009. Export of a Toxoplasma gondii Rhoptry Neck Protein Complex at the Host Cell Membrane to Form the Moving Junction during Invasion. Plos Pathogens, 5.
Blackman, M. J. & Bannister, L. H. 2001. Apical organelles of Apicomplexa: biology and isolation by subcellular fractionation. Molecular & Biochemical Parasitology, 117, 11-25.
Bouma, M. J., Smallridge, C. J., Bull, M. C. & Komdeur, J. 2006. Susceptibility to infection by a harmogregarine parasite and the impact of infection in the Australian sleepy lizard Tiliqua rugosa. Parasitology Research, 100, 949-954.
Brooks, C. F., Johnsen, H., van Dooren, G. G., Muthalagi, M., Lin, S. S., Bohne, W., Fischer, K. & Striepen, B. 2010. The Toxoplasma Apicoplast Phosphate Translocator Links Cytosolic and Apicoplast Metabolism and Is Essential for Parasite Survival. Cell Host & Microbe, 7, 62-73.
Carruthers, V. B. 1999. Armed and dangerous: Toxoplasma gondii uses and arsenal of secretory proteins to infect host cells. Parasitology International, 48, 1-10.
Cavalier-Smith, T. 1999. Principles of Protein and Lipid Targeting in Secondary Symbiogenesis: Euglenoid, Dinoflagellate, and Sporozoan Plastid Origins and the Eukaryote Family Tree. Journal of Eukaryote Microbiology, 46, 347-366.
Ciancio, A., Scippa, S., Finetti-Sialer, M., De Candia, A., Avallone, B., De Vincentiis, M. 2008. Redescription of Cardiosporidium cionae (Van Gaver and Stephan, 1907) (Apicomplexa: Piroplasmida), a plasmodial parasite of ascidian haemocytes. Eur J Protistol. 44(3):181-96.
Clopton, R. E. 2009. Phylogenetic relationships, evolution, and systematic revision of the septate gregarines (Apicomplexa: Eugregarinorida: Septatorina). Comparable Parasitology, 76, 167-190.
Cox, F. E. 2010. History of the discovery of the malaria parasites and their vectors. 3.
Dyson, J., Grahame, J. & Evennett, P. J. 1994. The apical complex of the gregarine Digyalum oweni (Protozoa: Apicomplexa). Journal of Natural History, 1994, 1-7.
Fast, N. M., Kissinger, J. C., Roos, D. S. & Keeling, P. J. 2001. Nuclear-encoded, plastid-targeted genes suggest a single common origin for apicomplexan and dinoflagellate plastids. Molecular Biology and Evolution, 18, 418-426.
Fast, N. M., Xue, L., Bingham, S. & Keeling, P. J. 2002. Re-examining Alveolate evolution using multiple protein molecular phylogeny. Journal of Eukaryote Microbiology, 49, 30-37.
Fernando, M. A. 1974. Fine structure of the schizonts and merozoites of Eimeria acervuina in the chicken. The Journal of Parasitology, 60, 149-159.
Green, B. R. 2011. Chloroplast genomes of photosynthetic eukaryotes. Plant Journal, 66, 34-44.
Grimwood, J. & Smith, J. E. 1996. Toxoplasma gondii: The role of parasite surface and secreted proteins in host cell invasion. International Journal for Parasitology, 26, 169-173.
Hamilton, W. & Zuk, M. 1982. Heritable true fitness and bright birds - A role for parasites. 218, 4570.
Horak, P., Saks, L., Karu, U., Ots, I., Surai, P. & McGraw, J. K. 2004. How coccidian parasites affect health and appearance of greenfinches. Journal of Animal Ecology, 73, 935-947.
Hu, K., Roos, D. S. & Murray, J. M. 2002. A novel polymer of tubulin forms the conoid of Toxoplasma gondii. Journal of Cell Biology, 156, 1039-1050.
Imam, T. S. 2009. The complexities in the classification of protozoa: A challenge to parasitologists. Bayero Journal of Pure and Applied Sciences, 2, 159-164.
Janouskovec, J., Horak, A., Obornik, M., Lukes, J. & Keeling, P. J. 2010. A common red algal origin of the apicomplexan, dinoflagellate, and heterokont plastids. Proceedings of the National Academy of Sciences of the United States of America, 107, 10949-10954.
Jirku, M., Valigurova, A., Koudela, B., Krizek, J., Modry, D. & Slapeta, J. 2008. New species of Cryptosporidium Tyzzer, 1907 (Apicomplexa) from amphibian host: morphology, biology and phylogeny. Folia Parasitologica, 55, 81-94.
Kawai, S., Igarashi, I., Abgaandorjiin, A., Miyazawa, K., Ikadai, H., Nagasawa, H., Fujisaki, K., Mikami, T., Suzuki, N. & Matsuda, H. 1999. Ultrastructural characteristics of Babesia caballi in equine erythrocytes in vitro. Parasitology Research, 85, 794-799.
Krotoski, W. A. 1985. Discovery of the hypnozoite and a new theory of malarial relapse. Transactions of the Royal Society of Tropical Medicine and Hygiene, 79, 1-11.
Lang-Unnasch, N., Reith, M. E., Munholland, J. & Barta, J. R. 1998. Plastids are widespread and ancient in parasites of the phylum Apicomplexa. International Journal for Parasitology, 28, 1743-1754.
Leander, B. S. 2007a. Marine gregarines: evolutionary prelude to the apicomplexan radiation? Trends in Parasitology, 24, 60-67.
Leander, B. S. 2007b. Molecular phylogeny and ultrastructure of Selenidium serpulae (Apicomplexa, Archigregarinia) from the calcareous tubeworm Serpula vermicularis (Annelida, Polychaeta, Sabellida). Zoologica Scripta, 36, 213-227.
Lee, J. J., Leedale, G. F. & Bradbury, P. (eds.) 2000. An illustrated guide to the Protozoa, Kansas: Society of Protozoologists.
Levine, N. D. 1973. The Apicomplexa and the coccidia proper. Protozoan parasites of domestic animals and man. 2nd ed. Minneapolis: Burgress Publishing Company.
Mackintosh, C. L., Beeson, J. G. & Marsh, K. 2004. Clinical features and pathogenesis of severe malaria. Trends in Parasitology, 20, 597-603.
Manguin, S., Bangs, M. J., Pothikasikorn, J. & Chareonviriyaphap, T. 2010. Review on global co-transmission of human Plasmodium species and Wuchereria bancrofti by Anopheles mosquitoes. Infection Genetics and Evolution, 10, 159-177.
Markus, M. B. 2010. The hypnozoite concept, with particular reference to malaria. Parasitological Research, 108, 247-252.
McFadden, G. I., Reith, M. E., Munholland, J. & LangUnnasch, N. 1996. Plastid in human parasites. Nature, 381, 482-482.
Moller, A. P. & Arriero, E. 2008. Host ecology and life-history traits associated with blood parasite species richness in birds. Journal of Evolutionary Biology, 21, 1504-1513.
Moore, R. B., Obornik, M., Janouskovec, J., Chrudimsky, T., Vancova, M., Green, D. H., Wright, S. W., Davies, N. W., Bolch, C. J. S., Heimann, K., Slapeta, J., Hoegh-Guldberg, O., Logsdon, J. M. & Carter, D. A. 2008. A photosynthetic alveolate closely related to apicomplexan parasites. Nature, 451, 959-963.
Morin-Adeline, V., Vogelnest, L., Dhand, N. K., Shiels, M., Angus, W. & Slapeta, J. 2011. Afternoon shedding of a new species of Isospora (Apicomplexa) in the endangered Regent Honeyeater (Xanthomyza phrygia). Parasitology, 138, 713-724.
Morrison, D. A. 2009. Evolution of the Apicomplexa: where are we now? Trends in Parasitology, 25, 375-382.
Prensier, G., Dubremetz, J. F. & Schrevel, J. 2008. The unique adaptation of the life cycle of the coelomic gregarine Diplauxis hatti to its host Perinereis cultrifera (annelida, Polycharta): an experimental and ultrastructural study. Journal of Eukaryote Microbiology, 55, 541-553.
Proellocks, N. I., Coppel, R. L. & Waller, K. L. 2010. Dissecting the apicomplexan rhoptry neck proteins. Trends in Parasitology, 26, 297-304.
Saffo, M.B., McCoy, A.M., Rieken, C., Slamovits, C.H. 2010. Nephromyces, a beneficial apicomplexan symbiont in marine animals. Proc Natl Acad Sci U S A. 107(37):16190-5.
Sharman, P. A., Smith, N. C., Wallach, M. G. & Katrib, M. 2010. Chasing the golden egg: vaccination against poultry coccidiosis. Parasite Immunology, 32, 590-598.
Shaw, M. K. 2003. Cell invasion by Theileria sporozoites. Trends in Parasitology, 19, 2-6.
Trees, A. J., Davison, H. C., Innes, E. A. & Wastling, J. M. 1999. Towards evaluating the economic impact of bovine neosporosis. International Journal for Parasitology, 29, 1195-1200.
Van de Peer, Y. & De Wachter, R. 1997. Evolutionary relationships among the eukaryotic crown taxa taking into account site-to-site rate variation in 18S rRNA. Journal of Molecular Evolution, 45, 619-630.
Waller, R. F. & McFadden, G. I. 2005. The Apicoplast: A Review of the Derived Plastid of Apicomplexan Parasites. Current Issue of Molecular Biology, 7, 57-80.
Williamson, D. H., Preiser, P. R., Moore, P. W., McCready, S., Strath, M. & Wilson, R. J. M. 2002. The plastid DNA of the malaria parasite Plasmodium falciparum is replicated by two mechanisms. Molecular Microbiology, 45, 533-542.
Wilson, R. J. M., Denny, P. W., Preiser, P. R., Rangachari, K., Roberts, K., Roy, A., Whyte, A., Strath, M., Moore, D. J., Moore, P. W. & Williamson, D. H. 1996. Complete gene map of the plastid-like DNA of the malaria parasite Plasmodium falciparum. Journal of Molecular Biology, 261, 155-172.
Zhu, G., Marchewka, M. J. & Keithly, J. S. 2000. Cryptosporidium parvum appears to lack a plastid genome. Microbiology-Uk, 146, 315-321.
Title Illustrations

Scientific Name | Monocystis sp. |
---|---|
Comments | Monocystis sp. in the seminal vesicles of common earthworm. |
Specimen Condition | Live Specimen |
Image Use |
![]() |
Copyright |
© Jan Šlapeta
![]() |
Scientific Name | Isospora lesouefi |
---|---|
Comments | Oocyst of Isospora lesouefi under bright light and UV light. |
Specimen Condition | Live Specimen |
Image Use |
![]() |
Copyright |
© Jan Šlapeta
![]() |
Scientific Name | Theileria ornithorhynchi |
---|---|
Comments | blood of a platypus with Theileria ornithorhynchi |
Image Use |
![]() |
Copyright |
© Jan Šlapeta
![]() |
About This Page
This page is being developed as part of the Tree of Life Web Project Protist Diversity Workshop, co-sponsored by the Canadian Institute for Advanced Research (CIFAR) program in Integrated Microbial Biodiversity and the Tula Foundation.
Jan lapeta
Faculty of Veterinary Science, University of Sydney, New South Wales, Australia
Victoria Morin-Adeline
Faculty of Veterinary Science, University of Sydney, Australia
Correspondence regarding this page should be directed to Jan Šlapeta at
Page copyright © 2011 Jan Šlapeta and Victoria Morin-Adeline
Page: Tree of Life
Apicomplexa
Authored by
. Sporozoa .Jan Šlapeta and Victoria Morin-Adeline.
The TEXT of this page is licensed under the
Creative Commons Attribution-NonCommercial License - Version 3.0. Note that images and other media
featured on this page are each governed by their own license, and they may or may not be available
for reuse. Click on an image or a media link to access the media data window, which provides the
relevant licensing information. For the general terms and conditions of ToL material reuse and
redistribution, please see the Tree of Life Copyright
Policies.
- First online 28 April 2008
- Content changed 18 May 2011
Citing this page:
lapeta, Jan and Victoria Morin-Adeline. 2011. Apicomplexa http://tolweb.org/Apicomplexa/2446/2011.05.18 in The Tree of Life Web Project, http://tolweb.org/
. Sporozoa . Version 18 May 2011.